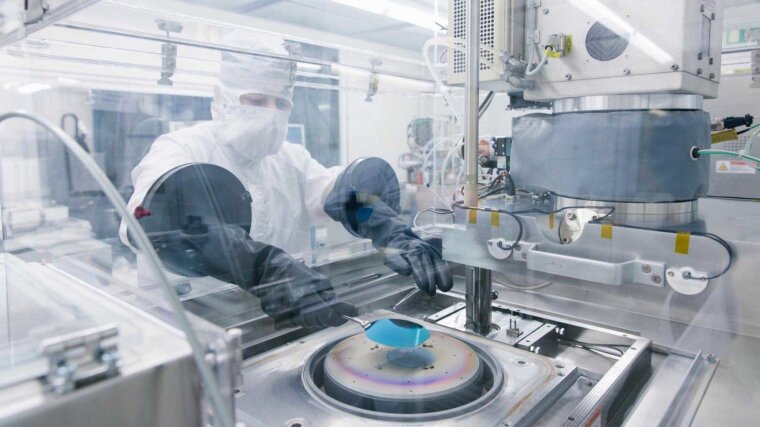
Until now, scientists have not been able to directly observe what happens between individual atoms and molecules when they react with one another. The signals generated by the interaction between light, atoms and molecules are too weak to be detected by conventional light-based methods. However, a team of researchers based at the University of Jena have developed a strategy to enhance the weak signals with plasmonic and nonlinear optical effects, which is the first step towards being able to directly observe chemical reactions in individual molecules.
By Ute Schönfelder
We bring secrets ›to light‹. We want to ›shed light‹ on the nature around us. When we have an idea, ›a lightbulb goes off in our head‹ … There are countless metaphors about the enlightening effect of light – and for good reason: »If we want to learn something about our environment, the matter and molecules around us and the fundamental processes occurring within them, we need light,« says Prof. Dr Jürgen Popp, a chemist at the University of Jena. »Light is the key to what we see, from the simplest of microscopes (like the ones we remember from school) to state-of-the-art imaging processes based on interactions between laser light and matter«.
Popp and his teams at the Institute of Physical Chemistry of the University Jena and the Leibniz Institute of Photonic Technology (Leibniz-IPHT) are researching spectroscopic methods that might enable us to take a closer look at a chemical reaction.
To do this, they are optimizing Raman spectroscopy (see box below), an analytical form of spectroscopy with a wide range of applications, such as the analysis of drinking water and foodstuffs or the clinical detection of pathogens. Raman spectroscopy is a contactless method that can be used to uniquely identify any material or molecule. »The spectrum of a sample is like a chemical fingerprint,« highlights Prof. Dr Michael Schmitt, who is a member of Popp’s team.
However, the Raman signal, which is based on an inelastic scattering of light, is a very weak process; if the researchers want to look at individual molecules, the Raman signals therefore have to be enhanced to be able to measure them at all.
And that’s exactly what Jürgen Popp and Michael Schmitt are working on together with PD Dr Jer-Shing Huang from the Leibniz-IPHT at the ›Nonlinear Optics down to Atomic Scales‹ collaborative research centre. The researchers have just made a first important step: in a paper published in ›ACS Nano‹ they combine two methods with partners from Taiwan to effectively enhance the weak Raman signal.
Plasmonic enhancement of weak Raman signals
On the one hand, they are using plasmonic nanostructures as optical nanoantennas to concentrate light onto even nanometre-sized areas and thereby increasing the interaction between light and matter. In this case, the researchers are using plasmonic structures to enhance the weak Raman signal: The electrons in the nanostructures are excited by a laser to form so-called surface plasmons, thus creating a strong electrical field with which molecules absorbed at the hotspots can interact much effectively with light. This intensifies the interaction between the Raman excitation light and the molecules examined, thus increasing the intensity of the Raman scattering effect. This method, which is known as ›surface-enhanced Raman scattering‹ (SERS), can be used to enhance the Raman signal by orders of magnitude compared with normal Raman spectroscopy.
The team of researchers are conducting their experiments with nanostructures made of gold, which are cut into highly smooth, individual crystals known as ›gold flakes« which are around 300 nanometres thick. »We’re using different shapes and sizes of nanostructures, as we want to find out how the design affects the plasmonic enhancement effect,« explains Jer-Shing Huang.
Schematic representation of the nanoplatform used to enhance Raman spectroscopy: Both the excitation rays (green and red) and the Raman scattering (blue) are coherently bundled by the ring-shaped nanostructures. This coherent bundling is further enhanced by the surface plasmons in the coloured areas, which interact with the examined molecules (spherical models) to increase the intensity of the Raman signal by twelve orders of magnitude.
Image: Liana Franke/Uni JenaGold nanostructures act as antennas for laser light
The nanotechnology expert and his colleagues are taking a targeted approach. Theoretical groups led by Prof. Dr Stefanie Gräfe and Prof. Dr Ulf Peschel from the collaborative research center first model the interaction of the structures with light on the computer in order to derive the optimum design parameters for the desired effect. In addition to the SERS method, the study published by the researchers from the University of Jena and Leibniz-IPHT also features another way to enhance the weak Raman signal: by utilizing nonlinear light matter interactions the Raman scattered light excited by intense short-pulse lasers is coherently bundled. This method, known as ›coherent anti-Stokes Raman scattering‹ (CARS), also results in enhanced Raman signals. In their recent publication, the researchers created a gold surface structured with nanoscale rings (see figure aside) and used this to plasmonically enhance both the incident laser light and the coherent Raman signal. The resulting process, known as ›nonlinear surface-enhanced coherent anti-Stokes Raman scattering‹ (SECARS), combines the enhancement mechanisms of both CARS and SERS to enhance the Raman signal by up to twelve orders of magnitude.
The researchers concluded that their findings could be used to considerably shift the limit of detection for Raman spectroscopy. »In addition to the existing advantages of the process, such as the fact that sample molecules can be used directly without the need for dyes, the method is now highly sensitive,« explains Jürgen Popp. Michael Schmitt adds that the aim is to refine the method to the point where chemical reactions can be directly observed in individual molecules: »every chemist’s dream«.
Raman spectroscopy
In 1928, the Indian physicist Chandrasekhara Venkata Raman, who would go on to win the Nobel Prize, discovered the ›Raman effect‹: When monochromatic light particles hit molecules, the light is scattered. While most of the light retains its wavelength and frequency (i.e. the scattering is elastic), approximately every millionth photon is scattered inelastically (i.e. energy is transferred from the light field to the molecule or from the molecule to the light field). In each case, the scattered photons have a different wavelength (frequency, energy) to that of the incident light.
This inelastically scattered radiation is used in Raman spectroscopy, where oscillation energy is transferred; as this energy is unique to each atom or molecule, Raman spectra are like chemical fingerprints that provide unique information about the composition of the sample examined.
Original-Publication:
Spatially Resolving the Enhancement Effect in Surface-Enhanced Coherent Anti-StokesRaman Scattering [...], ACS Nano 2021, DOI: 10.1021/acsnano.0c07198External link
07743 Jena Google Maps site planExternal link